Drs. Dave Schriemer and Susan Lees-Miller to Study DNA double-strand breaks
Dr. Dave Schriemer in collaboration with Dr. Susan Lees-Miller will use integrative methods in structural biology to determine how two major pathways for repairing DNA double-strand breaks (DSBs) converge on a master end-sensing synapse.
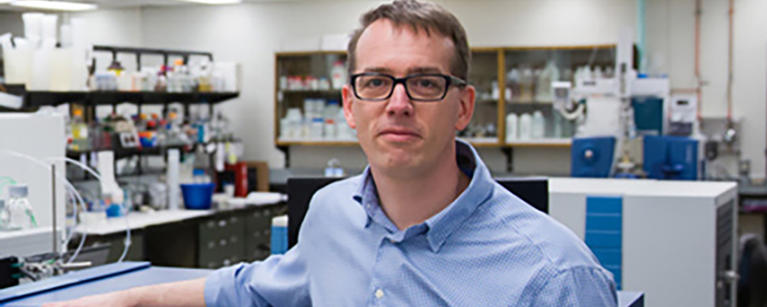
Congratulations to Dave Schriemer and Susan Lees-Miller on their Canadian Institutes of Health Research (CIHR) grant to Study DNA double-strand breaks
Prepared by Dr. Dave Schriemer
This project will use integrative methods in structural biology to determine how two major pathways for repairing DNA double-strand breaks (DSBs) converge on a master end-sensing synapse. We seek to understand how conformational changes in DNA-PKcs (a key element of the complex) controls pathway progression. The work has fundamental value in understanding the mechanism of a critical cellular process. It will uncover new strategies for sensitizing cancer cells to radiotherapy and genotoxins, through allosteric regulation of this important drug target.
Ionizing radiation and many chemotherapeutic drugs act by creating DSBs in order to overwhelm cellular mechanisms of DNA repair. A cascade of repair pathways attempts restoration of the genome across all cell stages, but with varying degrees of fidelity. Two canonical pathways, non-homologous end-joining (NHEJ) and homologous recombination (HR), process most DSBs with good outcomes and until recently pathway routing was seen to have a strong kinetic component, where the fastest repair mechanism dominates (NHEJ). However, the initiation machinery for both pathways is recruited to DSBs near simultaneously, in what appears to be a master end-sensing synapse dominated by the ultra-large kinase DNA-PKcs. The kinase occupies the terminal position at break, which we have shown places the ends in a surprisingly well-protected pocket. DNA-PKcs is a gate-keeper of repair, but it is not at all clear how the kinase coordinates the execution of NHEJ from this position while at the same time acting as a brake on the HRR initiation machinery. This mechanistic uncertainty contributes to the general failure to exploit this kinase in cancer therapy. We will use crosslinking mass spec, hydrogen/deuterium exchange mass spec and cryo-electron microscopy together with integrative modeling to determine the structure-function properties of the kinase as it engages mimics of DSBs.
Our hypothesis is that DNA-PKcs establishes an end-sensing synapse to control NHEJ progression and regulate resection. We propose that control is exerted through a series of induced conformational transitions, ultimately leading to the expulsion of DNA-PKcs when “dirty” DNA breaks are sufficiently “cleaned” by a nuclease, to allow repair to proceed. To test this hypothesis, we will pursue the following distinct but complementary aims over a five-year period:
1. Use HX-MS and cryo-EM to evaluate how autophosphorylation coordinates with DSBs to induce conformational transitions,
2. Apply XL-MS and integrative modeling to determine how DNA-PKcs builds an end-sensing complex and establishes the conditions for pathway progression, and
3. Use resection assays, XL-MS and integrative modeling to test how DNA-PK exerts a regulatory function on the MRN complex.
Almost 25 years of investigation have failed to clarify how DNA-PKcs regulates NHEJ mechanistically, because of the interrelatedness of repair pathways and the difficulty in controlling the chemical nature of the DNA damage in the cell. A structural solution is required, using overlapping methods that can accommodate the size, dynamics and disordered domains involved in regulation. Integrative structural methods have matured to the point where such a project is possible, providing us the first opportunity to observe how the repair of complex DSBs is executed, and in turn, our finding will teach us how to develop more selective ways to target tumors with radiotherapy.